What Does Success Look Like? Ensuring a Smooth Transition to the Next-Generation Power Grid
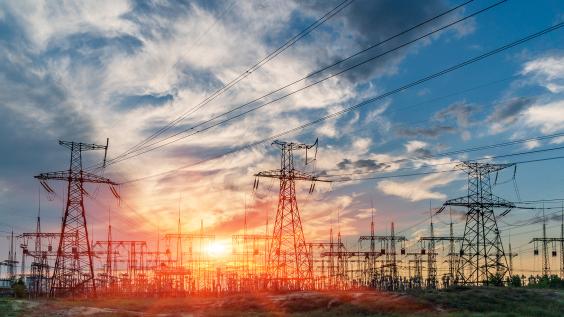
Table of Contents
Author(s)
Share this Publication
- Print This Publication
- Cite This Publication Copy Citation
Julie A. Cohn, “What Does Success Look Like? An Update on Efforts to Ensure a Smooth Transition to the Next-Generation Power Grid” (Houston: Rice University’s Baker Institute for Public Policy, June 12, 2024), https://doi.org/10.25613/GQAV-BN65.
An Evolving Power Grid in the 21st Century
Today, we are in the midst of a rapid transition from the 20th-century power grid — dominated by giant spinning generators that connect directly to transmission lines — to a 21st-century power grid replete with wind and solar farms, energy storage units, rooftop solar, electric vehicles, and myriad other devices that connect via power electronics. For many in the United States and elsewhere, the goal is a net-zero or low-carbon grid powered by renewable energy sources to meet our growing electricity demands.
The power electronics used to link renewables to the grid are called inverters, and they are fundamentally different from traditional spinning generators. Consequently, the current transition is not only a shift from fossil-fuel-based resources to renewables, but also a shift from physics-based control to electronics-based control.
Ensuring the stability, reliability, and resilience of an electronics-controlled power grid remains a challenge, but numerous groups are working toward these goals. At the February 2024 meeting of one of these organizations, the Universal Interoperability of Grid-forming Inverters (UNIFI) Consortium, project leader Ben Kroposki posed the question, “What does success look like?” He answered, “When the technologies are deployed, they all work, and no one wonders how we got here.”
Kroposki, who works at the National Renewable Energy Laboratory (NREL), leads a collaborative team of academic researchers, manufacturers, utilities, regulators, and system operators who are halfway through a Department of Energy funded five-year project to aid this transition. For his team, success will look like the smooth integration of renewable energy sources without disrupting grid operations, the seamless grid connection of devices from competing manufacturers, and the absence of noticeable differences in power quality or availability for all customers, perhaps with improvements compared to current standards.
This issue brief examines recent developments and historical analogues relevant to achieving this vision of a successful power grid transition.
How Grid-Forming Inverters Could Be a Game Changer
Our current electric power grid is predominately comprised of giant machines with spinning turbines, such as those found in hydroelectric, nuclear, gas-fired, and coal-fired power plants. As a matter of physics, the voltage and alternating current from these generators provide information that we rely on to control the flow of power on the grid.
In contrast, solar arrays and energy storage facilities generate direct current, which must be converted to alternating current by power electronic devices before reaching the grid. While wind turbines generate alternating current, it is first rectified into direct current, then converted again by power electronic devices into alternating current for use on the grid. Known as inverters, these devices are, for the most part, programmed to emulate traditional generators by following the voltage and frequency of the grid to determine whether renewable sources should inject power. Consequently, they are called grid-following (GFL) inverters.
However, grid operators have discovered that GFL inverters pose challenges under certain conditions — particularly in places with a high penetration of grid-following inverter-based resources. There is also a lack of understanding about how the grid will function once the number of GFL inverters exceeds a certain threshold. In fact, researchers don’t even know what that threshold might be!
Consequently, the UNIFI Consortium is exploring an alternative approach: grid-forming (GFM) inverters, which are programmed to provide local voltage and frequency references to the grid and, in theory, could improve the stability and resilience of the power system.
Currently, dozens of manufacturers produce inverters and sell them to thousands of users. In the near future, millions of inverters will be integrated into the grid. UNIFI is exploring ways to ensure that this multitude of inverters work and play well together. This can be a challenge since inverters are essentially black boxes controlled by proprietary software.
As a historian who has studied the evolution of today’s grid — an incomprehensibly large and complex machine that is stable and reliable most of the time — I do want to understand how we may arrive at that successful future.
Despite Promise of Grid-Forming Inverters, Concerns Persist
Last summer, at the consortium’s second General Meeting in Orlando, Florida, UNIFI participants offered both optimistic and cautionary status reports. Various projects across the power industry used digital simulations to explore the potential of GFM inverters in relatively simple systems, with investigators reporting several successes. However, three primary concerns emerged:
- Can engineers break out of their silos sufficiently to communicate in a common language about this energy transition?
- Can engineers develop a “middle layer” — i.e., a framework to allow consistent operation and validation of models and devices, regardless of the proprietary nature of the inverters?
- How can simulations, models, and resulting specifications assure that the inverters will be truly interoperable once connected to the grid?
At the 2024 Annual Meeting in February, researchers offered updates on these issues. Specifically, consortium leaders organized a number of cross-cutting teams intended to increase communication and collaboration across different research areas. These teams are working to develop a common language as they design the “middle layer” and run tests to determine whether multiple GFM inverters, despite their proprietary designs, can achieve interoperability.
Existing Projects Highlight Both the Potential and Challenges of Integration
UNIFI researchers also reported on projects already underway, further elucidating the complications and solutions introduced with GFM inverters, the challenges posed by GFL inverters, and the tendency of GFM inverters in certain arrangements to “fight.” Several of these projects are described below.
GFM Alleviates Loss of a Kauai Power Plant
The Hawaiian island of Kauai has a power grid dominated by inverter-based resources, mostly grid-following. In 2021, the island’s largest power plant experienced a trip. Although storage batteries provided sufficient power to avert an outage, the system did experience oscillations related to four inverters — three GFL inverters and one GFM inverter.
Researchers then simulated the event with alternative programming to understand how the system might respond with more GFM inverters. By spring of 2023, one of the GFL inverters was upgraded to a GFM inverter, and when the same power plant tripped again, the system experienced no oscillations.
Grid Weakness With Texas GFL Inverters
The Electric Reliability Council of Texas (ERCOT), which operates the Texas power grid, recorded problems in the Odessa region due to GFL inverters in 2020 and 2021. Following these issues, engineers simulated operations of the West Texas region that demonstrated how the system, enhanced by GFM inverters, would perform under various scenarios. The findings indicated that integrating GFM inverters could create significantly more stable grid conditions. However, it was also noted that GFM alone would not address all potential problems in the area.
Problems With Parallel GFM in German Field Test
Ulrich Muenz, representing Siemens Technology from Germany, reported on a field test using multiple GFM inverters. In this test, all the generators and batteries were connected to a system via these inverters. It was observed that, under certain scenarios, GFM inverters operating in parallel became unstable. Muenz described the inverters as “fighting” against each other before disconnecting. He emphasized the need to better understand how and where to deploy GFM inverters.
Looking at History to Address Today’s Challenges
One of the most exciting initiatives described by a cross-cutting team at the UNIFI meeting involved side-by-side tests of GFM inverters from multiple manufacturers on a model system.
These types of side-by-side tests and concerns over fighting devices are not new and have, in fact, occurred throughout the 20th century. In 1928, an engineer reported to his company’s Development Committee that “if there is a discrepancy between the two controllers, there would almost certainly be a tendacy for the two instruments to ‘fight.’”[1] The prior year, the engineer’s company, Leeds & Northrup, had participated in a field test with a competing device from General Electric Company. The two frequency control devices were installed side by side on a small interconnected system, and the results revealed better frequency stability regardless of which device was used. This highlighted the overall benefits of automatic frequency control, but the test also revealed that the devices interfered with scheduled load distribution, indicating the need for further research.
Such field tests and “fights” continued throughout the next few decades as electricity companies grappled with one of the most significant conundrums of the mid-20th-century grid: how to maintain stable operations on interconnected power systems (see Figure 1). In the 1920s and 1930s, in fact, utility managers lamented that there were no controls that ensured stability while simultaneously respecting the contracts between linked companies. Both well-known and lesser-known manufacturers, such as General Electric, Westinghouse, and Leeds & Northrup Company, competed fiercely to gain the business of power companies. With their proprietary inventions — and a litigious history — they were reluctant to cooperate. Yet the only way the industry came to understand how to control alternating current power on interconnected systems was through collaboration.
Figure 1 — Image of Southern California Edison Control Room Showing Clocks and Meters From Multiple Vendors on Right-Hand Side of Wall, ca. 1930

Companies had been building interconnections since the beginning of the 20th century. The power-sharing agreements ranged from handshakes to formal contracts, and by the 1920s, fairly significant networks appeared across the country (Figure 2). Interestingly, the electricity on these networks responded more reliably to the laws of physics than the laws of the market. Regardless of which company planned to sell power to which customer, all the generators on the interconnection responded to changes in demand nearly simultaneously. In other words, when someone started up the conveyor belt at a factory, or a power line tripped off the grid, every generator “felt” the change, and responded to it. Not only did the human system operators have to make manual adjustments on a minute-by-minute basis, but the contractual agreements regarding power sales often did not align with actual power distribution. As the saying goes, electricity “followed the path of least resistance” (and impedance).
Figure 2 — Map Illustrating Extent of Transmission and Interconnection Development by 1928

Therefore, inventors, manufacturers, academics, and utility engineers began producing proprietary methods and devices to automatically control the flow of electricity on these networks. To learn whether a control instrument could do the job, the inventor had no choice but to install it on the power system and give it a try, while real people were busy turning things on and off, hopefully unaware of the experiment underway. It was not uncommon for a utility to install competing devices side by side to compare how they worked.
These tests were not always friendly. In 1931, competitors Leeds & Northrup Company and General Electric again agreed to place their devices on the same system, this time in Ohio. Participating utilities, the National Electric Light Association (the forerunner of today’s Edison Electric Institute), and others observed the test. The competitors, though friendly at the start, sniped at each other. For example, a General Electric engineer stayed on site full time to monitor and help with the test. Leeds & Northrup saw this as an underhanded attempt to gain “all the advantages,” and decided to “play ball” and do the same.[3]
In the end, the results illustrated that centralizing control at one facility was burdensome, while fully distributing control without standardized settings on the devices was problematic. Though ultimately inconclusive, the results did point to what success could look like: a combination of distributed and centralized control that respected the boundaries of each operating system and adhered to the contractual agreements in place.
Making the Leap From Simulations to Real-Time Operations
Today, while the technologies and circumstances are substantially different, organizations like UNIFI are once again confronting challenges similar to those faced a century earlier. Reflecting on discussions from the UNIFI Annual Meeting, if the inverters involved in the German field test were indeed “fighting,” one wonders how UNIFI’s middle layer will ensure that devices from multiple manufacturers will work and play well together. Some 21st-century engineers express admiration for the courage of their forebears to experiment directly on the grid. Many also appreciate the flexibility, verisimilitude, and relative safety of the models run on today’s high-speed computers. Nonetheless, the leap from models and simulations to practical implementation on the grid remains a significant challenge.
UNIFI’s 1 megawatt (MW) multivendor test, underway this year, may be another stepping stone toward a successful grid transition. At the February meeting, NREL engineer Jing Wang explained that the test includes inverters from five different manufacturers, with their proprietary designs intact (see Figure 3). Prior to the meeting, I asked Wang if it was unusual for competing manufacturers to participate in an experiment like this. She thought it might be. Each of the inverters her team selected for the test is meant to meet the UNIFI-developed specifications for interoperability, but details of the internal designs are unknown. A system controller will dispatch the GFM inverters, which will all connect to the same testing circuit and follow the same testing protocols. Following this, UNIFI plans to scale up to a 20 MW demonstration, and then real-world demonstrations on systems larger than 20 MW.[4] While the results will reveal the interoperability of devices offered by five manufacturers, the test itself will demonstrate to both industry and academic researchers how they can collaborate to develop practical guidance for the physics-to-electronics transition underway.
Figure 3 — Engineers Monitor Frequency, Voltage, Active, and Reactive Power of Grid-Forming and Grid-Following Inverters and One Diesel Generator During 1 MW System Demonstration at NREL

Will Cooperation be the Key to Success?
In the United States, the experience of using electricity is nearly seamless. For the most part, the machines and devices providing electricity do not “fight.” Nor do the manufacturers, though they might compete. Perhaps in the policy arena, we will see some fighting. But the lessons from the past, and the experience that is unfolding at UNIFI right now, show that while fighting may illuminate the problems, cooperation will reveal the solutions. Indeed, contention and collaboration often go hand-in-hand.
In the end, to answer Kroposki’s original question — “What does success look like?” — I believe success will include specifications and standards that protect intellectual property, support commerce, and maintain reliable power system operations. When UNIFI achieves this, and the technologies are successfully integrated and interoperable, future historians should still be asking, “How did we get here?” Undoubtedly, the answer is going to be interesting.
Notes
[1] The spelling “tendacy” instead of “tendency” is as the source material reads (Development Committee Miscellaneous Report 180, 1911–39, Reel 7, Leeds & Northrup Records [1110], Hagley Museum and Library, Wilmington, DE,
https://findingaids.hagley.org/repositories/3/resources/860#).
[2] Report on the Status of Interconnections and Pooling of Electric Utility Systems in the United States, Edison Electric Institute, New York, 1962.
[3] S.B. Morehouse Memoranda to L. Heath, November to December, 1931, Leeds & Northrup Records [1110], Hagley Museum and Library, Wilmington, DE, https://findingaids.hagley.org/repositories/3/resources/860#).
[4] As points of comparison, the Electric Reliability Council of Texas (ERCOT) operates a system with 145,000 MW of generating capacity, and the United States overall has more than 1,000,000 MW.
This material may be quoted or reproduced without prior permission, provided appropriate credit is given to the author and Rice University’s Baker Institute for Public Policy. The views expressed herein are those of the individual author(s), and do not necessarily represent the views of Rice University’s Baker Institute for Public Policy.